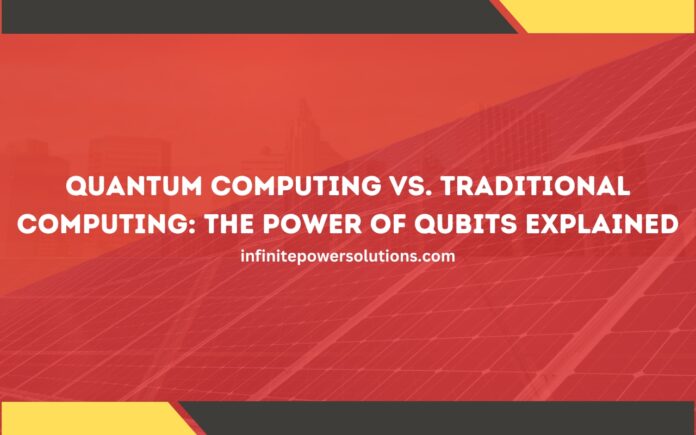
As technology continues to evolve at an unprecedented pace, a significant paradigm shift is happening in the realm of computing. The emergence of quantum computing promises to revolutionize how we process information, offering capabilities that traditional computing cannot. This article delves into the fundamental differences between quantum and traditional computing, exploring the power of qubits and the potential implications for various fields.
At its core, quantum computing harnesses the principles of quantum mechanics, which govern the behavior of subatomic particles. This approach enables quantum computers to perform calculations at speeds unattainable by classical machines. Traditional computers, which have served us well for decades, function on a binary system, utilizing bits as the fundamental unit of information. A bit can exist in one of two states: 0 or 1. This binary framework allows for a systematic and logical processing of data, but it also imposes limitations on the complexity of problems that can be efficiently solved.
Quantum computers, on the other hand, utilize quantum bits, or qubits. Unlike classical bits, qubits can represent a zero, a one, or both at the same time due to a unique phenomenon known as superposition. This property allows quantum computers to explore multiple solutions simultaneously, drastically improving efficiency for specific computations. When combined with another quantum principle called entanglement, where qubits become interlinked in such a way that the state of one can depend on the state of another, the computational potential of quantum systems is further amplified.
The implications of quantum computing extend far beyond speed. This technology has the potential to tackle complex problems that are currently beyond the reach of classical computing, from optimizing logistics to simulating molecular interactions in drug development. As industries begin to recognize the transformative power of quantum computing, investment in research and development continues to grow, with estimates suggesting that the quantum computing market could reach USD 1.3 trillion by 2035, signaling a significant shift in how we approach problem-solving and data processing.
The Basics of Quantum Mechanics
To understand the power of qubits and the fundamental differences between quantum and traditional computing, it is vital to explore some key principles of quantum mechanics. Quantum mechanics describes the behavior of matter and energy at the smallest scales, where classical physics begins to break down. At this level, particles can exhibit behaviors that seem counterintuitive, such as being in multiple states at once or instantaneously affecting one another regardless of distance.
Superposition
Superposition is a cornerstone concept in quantum computing. When a qubit is in a state of superposition, it can simultaneously represent both a 0 and a 1. This is akin to flipping a coin—while it spins in the air, it is neither heads nor tails but rather a mixture of both outcomes. This property allows quantum computers to handle vast amounts of information and perform multiple calculations simultaneously. For instance, two qubits in superposition can represent four different states simultaneously, three qubits can represent eight states, and so forth. The ability to explore so many possibilities concurrently opens the door to solving complex problems at an exponential scale.
Entanglement
Entanglement is another fundamental principle of quantum mechanics that enhances the power of quantum computing. When qubits become entangled, the state of one qubit becomes dependent on the state of another, no matter the distance between them. This means that measuring one qubit instantly reveals information about its entangled counterpart. This phenomenon allows quantum computers to perform coordinated computations more efficiently than classical systems, significantly enhancing their processing power.
Decoherence and Quantum Error Correction
Although the promise of quantum computing is immense, it is not without challenges. Decoherence refers to the loss of quantum coherence, where qubits transition from their quantum state to classical states due to interference from their surroundings. This can lead to errors in computations. Overcoming decoherence is vital to the advancement of quantum computing. Researchers are actively working on quantum error correction methods to ensure the integrity of computations in quantum systems, which is crucial for achieving reliable and practical quantum computing solutions.
Comparing Quantum and Traditional Computing
The differences between quantum and traditional computing extend beyond the basic principles governing their operation. Understanding these differences sheds light on the unique advantages quantum computing offers.
Processing Power and Speed
Traditional computers process data in a sequential manner, executing one operation at a time, which often results in lengthy computation times for complex problems. In contrast, quantum computers can perform multiple calculations concurrently due to their ability to operate in superposition. This capability allows them to solve certain problems exponentially faster than classical computers. For example, tasks such as integer factorization, which is essential for encryption algorithms, would require an impractical amount of time for classical computers but can be tackled efficiently by quantum systems using algorithms like Shor's Algorithm.
Complexity of Problems
While classical computers excel at everyday tasks—such as word processing, internet browsing, and managing spreadsheets—they struggle with problems involving large datasets or intricate variables. Quantum computers, however, thrive in these areas. Their ability to represent complex states and relationships between data points allows them to approach problems in innovative ways. For instance, in optimization problems, such as supply chain management and route planning, quantum computers can evaluate multiple scenarios simultaneously, significantly reducing the time required to identify optimal solutions.
Types of Algorithms
The algorithms used in quantum computing are fundamentally different from those in classical computing. Quantum algorithms exploit quantum phenomena to achieve speedups in various computations. Notable algorithms include Shor's Algorithm for factoring large numbers and Grover's Algorithm for searching unsorted databases. These algorithms demonstrate the potential of quantum computing to outperform classical methods for specific tasks, showcasing how quantum principles can be harnessed for practical applications.
Applications Across Industries
The potential applications of quantum computing span numerous industries and fields. In finance, quantum computers could optimize investment portfolios and enhance risk assessment models, leading to more effective trading strategies. In healthcare, they hold promise for drug discovery by simulating molecular interactions at unprecedented levels of detail, potentially accelerating the development of new therapies. Additionally, in logistics, they can optimize supply chains, reducing costs and improving efficiency.
Moreover, quantum computing could revolutionize data security. While quantum computers pose a threat to traditional encryption methods, they also offer the opportunity to develop new, quantum-resistant algorithms that ensure data integrity in the quantum era. This duality presents a unique challenge that industries must navigate as they embrace this transformative technology.
The Road Ahead: Challenges and Opportunities
Despite the exciting prospects of quantum computing, it faces significant challenges that must be addressed to realize its full potential. Scaling quantum systems, improving qubit fidelity, and developing effective quantum error correction methods are just a few of the hurdles researchers are working to overcome.
Scaling Quantum Systems
To achieve practical quantum computing, researchers must develop systems that can maintain a large number of qubits. Current quantum computers are limited in the number of qubits they can effectively manage, which constrains their computational power. Plans are underway to scale quantum processors, such as IBM's vision for a quantum system with 200 logical qubits capable of running 100 million quantum gates by 2029. Achieving this level of scaling is paramount for unlocking the broader applications of quantum technology.
Improving Qubit Fidelity
Error rates and qubit stability present another significant challenge. Qubits are susceptible to environmental interference, which can degrade their quantum state and impact computation accuracy. Ensuring high fidelity—greater than 99.99 percent—is essential for building reliable quantum systems. Researchers are exploring various qubit technologies, such as superconducting qubits and trapped ions, to enhance performance and stability.
The Importance of Collaboration
Collaboration among academia, industry, and governments will be critical in advancing quantum computing technology. As researchers work to develop innovative solutions to existing challenges, partnerships can facilitate knowledge sharing, funding opportunities, and the alignment of efforts to drive progress in this exciting field.
The Future of Quantum Computing
As quantum computing technology advances, it promises to reshape our understanding of computation and expand our capabilities in various domains. The potential for quantum computing to address problems that classical computers cannot is not just a theoretical concept; it is rapidly becoming a reality. With ongoing research and development, industries are poised to harness the power of qubits to unlock new solutions and drive innovation.
Conclusion
Quantum computing stands at the forefront of a computing revolution, poised to redefine how we process information. By leveraging the unique properties of qubits and the principles of quantum mechanics, this emerging technology has the potential to tackle complex problems that were previously deemed insurmountable. As we navigate this exciting frontier, the collaboration between researchers, businesses, and governments will be essential for overcoming challenges and maximizing the benefits of quantum computing.
The journey into quantum computing is only just beginning. With every advancement, we draw closer to a future where powerful quantum systems can transform industries, solve global challenges, and redefine the limits of our computational capabilities. As technology evolves, so too will our ability to understand and leverage the complexities of the quantum world, leading to a new era of innovation and discovery.
For more information on quantum computing, check out resources like IBM's Quantum Computing Overview and McKinsey's Insights on Quantum Technology. These publications provide deeper insights into the principles, challenges, and future potential of quantum technology, offering a comprehensive understanding of one of the most significant technological advancements of our time.